Leptons
Leptons also come in three generations; Electron & Electron neutrino, Muon & Muon Neutrino, and Tau & Tau Neutrino, each with their associated anti-particle. Muons and Taus are, essentially, identical to electrons except for their masses. Leptons are fundamental particles that do not combine into other particles. All the leptons have ½ Spin, and, while neutrinos carry no charge, the other three carry identical charge of -1. Electrons and the three neutrinos are inherently stable, and thus the types we commonly see around us. The muon and the tau are much heavier than the other leptons. When they are produced in particle accelerators, they decay very quickly into lighter particles
The existence of neutrinos was inferred from neutron decay. A neutron decays into a proton and an electron, but experimenters found that momentum was not conserved, so they postulated the existence of the neutrino, in fact an anti-neutrino, to carry away the excess momentum. Subsequently, it was found experimentally. Neutrinos hardly react at all with other particles, and in fact every second about 50 trillion (50,000,000,000,000) pass harmlessly thorough your body. Initially thought to have zero mass, and therefore travel at the speed of light, recent experiments have confirmed neutrino oscillation, which requires them to have mass. Research, as of August 2010, indicates that the minimum mass is 0.05 eV, while the sum of the three neutrino masses must be less than 0.28 eV. A 2014 result gives a combined maximum mass of 0.320 ± 0.081 eV. The electron neutrino is the lightest, then the muon neutrino, then the tau neutrino, but all three masses are similar.
In September of 2011, the media published many articles about CERN claiming they had found neutrinos traveled at faster than light speed. In fact, the OPERA experiment in Italy, that uses muon neutrinos generated by CERN, reported anomalies in their measurements and asked the scientific community for assistance. By the following year they reported finding two errors in the experiment; a clock oscillator that was ticking too fast, and an incorrectly attached fiber optic cable. Neutrinos have a small but non-zero mass so travel at speeds very close to that of light
Physics
-
Standard Model
Electron
The electron was discovered by the British physicist J. J. Thomson in 1897, for which, in part, he received the Nobel prize in 1906. Its anti-particle is the positron. Paul Dirac first identified the possibility that an electron could have a positive charge. Initially, he thought the proton could be some sort of "positive electron", but, persuaded by Robert Oppenheimer, he suggested a new particle he called the anti-electron in 1931. Carl D. Anderson discovered the positron in 1932,] for which he received the Nobel Prize in 1936. This was the first example of what came to be known as anti-matter, and when an electron and a positron collide, assuming they do not scatter, they annihilate each other, producing pairs of photons in the form of gamma rays. In chemical reactions, electrons are responsible for the chemical bonding of atoms by being exchanged or shared by atoms of the substance. Positrons are used in PET (positron emission tomography) scanners. They are produced from the decay of a radioactive tracer.
Muon
Like the positron, the American physicist Carl D. Anderson discovered muons in 1936. Essentially, they behave like a heavy electron, weighing about 200 times more than an electron, but with the same charge and spin. This means that they bend less when passing through a magnetic field, which is how Anderson first observed them. They were very unexpected, at the time, leading I. I. Rabi to come up with his famous quote: "Who ordered that?".
As described in the section on Relativity, Muons were used to demonstrate the time dilation effect. This occurred for the first time in 1941 in an experiment conducted on Mount Washington. When a cosmic ray proton collides with nuclei in the upper atmosphere, pions are created which decay very quickly into muons and neutrinos. The half life of a muon without relativistic effects would allow it to travel only about 660 meters (2,145 feet) before it, too, would decay, most often into an electron, an electron-antineutrino, and a muon-neutrino. . The time dilation extends its lifetime allowing it to reach the Earth's surface, where they can be detected deep underground. Being heavier than an electron, they can penetrate much deeper into matter.
Rarely, an anti-muon binds with an electron and form an atom known as muonium, in which the muon acts as the nucleus. The mean lifetime of a Muon is only about 2.2 micro-seconds, but this is a long time in unstable sub-atomic particles where only the free neutron has a longer mean lifetime.
Tau
The tau lepton, or tauon, was first identified by Martin Lewis Perl and his colleagues at the SLAC-LBL group in a series of experiments between 1974 and 1977. It is nearly 3,500 times heavier than an electron, and decays very rapidly in about 2.9×10-13 seconds. Because of their mass, tau particles are potentially very highly penetrating, but this is limited by their short half life, so would only have any significant penetration at energies in the thousands of TeV, thus travelling at a significant proportion of light sspeed.
Uniquely among leptons, due to its high mass, it can decay into hadrons; for example, it could decay into a quark, an antiquark, and a tau neutrino. Interestingly, if supersymmetry is found to be correct, there would be at least five Higgs bosons, two charged and three neutral, and the neutral Higgs would generally decay into tau leptons.
Electron Neutrino
Early understanding of beta decay suggested that a neutron decayed into a proton and an electron. Experimental evidence showed, however, that the energy, momentum, and angular momentum of thee neutron were not conserved. In 1930, Wolfgang Pauli proposed a light neutral particle to explain this anomaly. In fact, this was the anti-particle of the neutrino, the anti-neutrino. This neutrino, now known as the electron neutrino, was finally discovered in 1956 by Clyde Cowan and Frederick Reines. There was long debate about whether the particle was massless, like a photon, and only recently has it been established that it has a tiny mass of at least 0.05 eV.
Muon Neutrino
The muon neutrino was first hypothesized by in the early 1940s, and discovered in 1962 by Leon Lederman, Melvin Schwartz and Jack Steinberger for which they received the 1988 Nobel Prize.
Tau Neutrino
the DONUT collaboration at Fermilab announced the first observation of the tau neutrino in July 2000. This meant that all the Standard Model particles have been observed except the Higgs. In the DONUT experiment, the Tevatron accelerated protons to produce tau neutrinos via the decay of charmed mesons. The resulting particles passed through a number of magnets, and iron and concrete blocks, where it was hoped that the majority of spurious particles would be removed. The remaining particles in the beam then passed through sheets of nuclear emulsion which would show the tracks of any particle interactions. By tracing back the particle paths through the sheets of emulsion, it was possible to identify the tracks of neutrinos.
Neutrino Oscillation
A neutrino of a particular type, (electron, muon or tau neutrino) can change over time, as it travels, into a neutrino of a different flavor. It was the observation of neutrino oscillation that confirmed that neutrinos have a non-zero, albeit very small. mass. If the particles were massless, then they would travel at light speed. Any particle, like the photon, that travels at light speed, does not experience the passing of time. So; if a neutrino did not experience time, it would not have time to oscillate. If it does oscillate, it cannot travel at light speed, so must have some mass. Bruno Pontecorvo first suggested the oscillation mechanism in 1957. Since then, many experiments using different detector technologies have confirmed its existence. The OPERA experiment uses a beam of muon neutrinos produced at CERN that travels about 730 km to the detector in the Gran Sasso National Laboratory near Rome, Italy. In May 2010, they announced that they had found that a muon neutrino changed into a tau neutrino sometime during the 2.4 milliseconds it took the beam to travel from CERN to Gran Sasso.
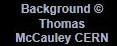